Pick up any tennis magazine or book and you'll find instruction
and advice on the full range of stroke production:
forehands, backhands, overheads, serves, volleys. You
name it - you can find information on technique and effective practices.
However, there haven't been many
biomechanic technology-based or scientific studies conducted
and published on footwork
in academic
journals.
Yet footwork is a major source of lost points in tennis.
You have to reach the ball in time to swing and
your body needs to be in a good biomechanical position to
strike the ball effectively. Being "off balance" or
stretching to reach the ball results in loss of power and
control. You may not be able to rotate your body
to obtain optimum power or control and place the ball in
your opponent's court effectively.
I'd like to share an interesting project on which two colleagues and I
collaborated. A few years back, we attempted a computer simulation of a tennis player.
The skeleton and full body model below are examples of that work.
On one of our visits with Dr. Paul Roetert at the USTA
(then the USTA's administrator of sport science, now
Managing Director
USA Tennis High Performance Program in Key Biscayne, FL), he told us
adding muscles to the skeleton would create a better tool.
Coaches, trainers and even players would be able to see
and understand the muscles activated during play. This could aid
in understanding why and how certain muscles are developed for tennis.
Adding muscles to the skeleton would be a big job,
but with support from the USTA our group was up for the task. We all wanted
to create
a model with a smoother and more realistic motion than the team's
previous models. In addition to skeletal motion, muscles "flex."
We decided that a "proof of concept" would be
developed. Instead of creating a new model for the entire
human body, the team would start with a smaller test
case. A general rule in research: if a technique
doesn't work for a simple case, it typically will not
work for a more complicated situation. Once the technique works
for a simpler case you can extend it to a more complicated one.
We felt the leg, from the kneecap to the
foot, would be ideal. Compared to other parts of
the body, leg motion is fairly limited in direction and range of motion.
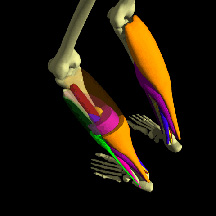
In The New Model Muscles Can be Cutaway To See The Underlying Muscles
And Skeleton
|
Compare the motion of your knee to the range of motion
of your wrist or your shoulder. The knee, wrist
and shoulder act as different types of joints. Your knee
acts like a hinge. Think of a door or cabinet
hinge. The door can open and close, but doesn't
operate in any other direction.
There are also different types of muscles. Bend your leg
at the knee and then straighten your leg to its original
position. Bending a joint is referred to as flexion;
straightening that bent limb is called extension. Muscles operate with
a pulling motion. Muscles do not push. In general
there is a muscle (or set of muscles) that
are used to bend joints and limbs - these are called
flexors. Different muscles, called extensors, are used to straighten the limb.
Unlike the knee joint, the wrist acts like a universal
joint (a combination of two hinges). While the door
hinge can move in one direction, the universal joint moves
back and forth in two directions. The shoulder has one
of the most complicated motions. It acts like a
ball-and-socket joint and can move and rotate in multiple directions.
There would be two major tasks in this part of the
footwork project: 1) create the new 3-dimensional
model and 2) capture a player's motion from the high speed video footage
and apply that motion to the 3D model.
Chad Okamoto, our company's graphic artist and animator created a new
and more realistic skeleton from the hips down. All major
leg muscles were added to the skeleton, from the knee
to the ankle. Each muscle was drawn in a different
color to make the muscles easier to see. The muscles
were given a flexing motion.
Links to 6 movies of the 3D model are listed here.
Next to the
link is the file size and file format .mov or .mpg.
You might want to download and save the files on your
computer and use them with your computer's movie player software.
The motion may look smoother on your own computer versus
the web. Set your movie player to "play all frames" and
you can use the "loop" feature
on your movie player to repeat the motion.
If you view the movie from the web try using
the "frame by frame" feature on the
movie player to make sure you get all the frames.
Have you ever heard the expression, "learn to walk before you run"?
We did the same with our model. Initially, our animator
had the model stand on its toes. This foot flex
was a precursor to the "unweighting" motion from the
ready position in tennis. Next a walking motion was applied to the model.
Even the
kneecap slides over the other leg bones when bending - you
can see this in the walking simulation.
Keep in mind that this is a 3D model versus 2D
animations. In 2D animation you draw (or have the
software draw) the different frames of the animation. In
2D animation when your character needs to turn from back to
front, you must draw the character's back and front.
Unlike the 2D animation, we only have to build the
3D model once. If we need to see the back
of the character, the 3D model is turned so that
you can see its back. Instead of drawing the character's
action, different motion files can be applied to the 3D
model. It's more like a computer marionette.
The marionette is a 3D computer generated model and different motions
are choreographed for the model to perform.
After the first model was done,
USTA sport science staff and committee members
reviewed the
model. They asked if
something could be done to the model to make it more obvious
that the muscles were flexing. The model was modified
so that the muscle color would intensify as the muscles shorten.
In the meantime, project staff captured a computer data file
containing information on the motion for each joint. Using
high speed footage
from the US Open, different joint positions were
recorded at each time step during the player's stroke. This
motion file was applied to the 3D model.
Although the original footage was shot facing the player from the
opposite court, with a 3D model we can create a
view from behind the player.
The model can be spun around, zoomed in
or out of and viewed from any angle. It can
even be cutaway to show the underlying muscles.
Everyone was quite pleased with the results.
Many Thanks: I'd like to acknowledge the USTA Sport Science Committee for
their support of this project
through a grant and Nick Saviano, Dr. Bill Aronson, Dr. Paul Roetert,
Dr. Duane Knudson and Marcie Londano for their input and support.
Cislunar Aerospace staff member Eric Chattot
did an excellent job of capturing the player motions from the footage.
Last, my friend and co-worker, the late Chad Okamoto did a wonderful job
creating the 3D model and I dedicate this column to him.
Until next month ... Jani